Measles
Ron Smith, MD
Measles in the News
This digitally colorized transmission electron micrographic (TEM) image reveals some of the ultrastructural morphology exhibited by both a paramyxovirus measles virus (MeV), and virions of the polyomavirus, simian virus SV40 at 40 nm.
Quick Overview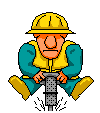
Measles Clinical Description
by Ron Smith, MD | August 19, 2022 | measleshistory | 0 Comments
Orenstein, Walter, et al. Plotkin’s Vaccines – Inkling Enhanced. Available from: Elsevier eBooks+, (7th Edition). Elsevier – OHCE, 2017.
Usual Clinical Course
The first symptoms of measles occur after a 10- to 14-day incubation period that follows wild measles virus exposure from a measles-infected person. A systematic review estimated the median incubation period to be 12.5 days (95% confidence interval [CI], 11.8–13.2) based on 55 observations from eight observational studies.48 Measles incubation periods vary by setting depending on population dynamics, measles virus transmission patterns during outbreaks, and the nature of infection events.49 For example, if infection occurs after parenteral exposure, the incubation period is shortened by 2 to 4 days. Individual case reports have suggested potential exposure events occurring at least 21 days prior to rash onset2,11,49–53; therefore, to ensure high sensitivity for disease surveillance, an incubation period of 7 to 21 days is often used for the purposes of conducting epidemiological investigations, and identifying contacts and potential transmission events to guide control activities.54 Immunosuppressed persons may have a prolonged incubation period because host immune responses, which are responsible for the signs and symptoms of measles, may be absent or delayed in persons with cellular immune deficiencies.55,56 The prodromal stage is heralded by the onset of fever, malaise, conjunctivitis, coryza, and tracheobronchitis (manifesting as cough) and lasts 2 to 4 days. This symptom complex is similar to that seen with any upper respiratory infection. The temperature rises during the ensuing 4 days and may reach as high as 40.6°C. Koplik spots, the enanthema believed to be pathognomonic of measles, appear as multiple 2- to 4-mm bluish-white plaques on the buccal mucosa, usually on the inside of the cheek opposite the first or second molar, 1 to 2 days before the onset of a rash and may be noted for an additional 1 to 2 days after rash onset.57–59 Koplik spots are believed to occur in greater than 70% of measles patients and because they usually first appear prior to rash onset, their early finding by a clinician during physical examination can prompt laboratory testing for rapid confirmation of measles, early treatment, implementation of infection-control practices, and postexposure vaccination of close contacts. Measles rash is an erythematous maculopapular eruption that usually appears 14 days after exposure and spreads from the head (face, forehead, hairline, ears, and upper neck) over the trunk to the extremities during a 3- to 4-day period. The exanthem is usually most confluent on the face and upper body and initially blanches on pressure. During the next 3 to 4 days, the rash fades in the order of its appearance and assumes a nonblanching brownish appearance.
Virus can be isolated from subcrevicular oral fluid, the nasopharynx, and blood during the latter part of the incubation period and during the early stages of rash development.8,60 Although virus has been isolated from the urine as late as 4 to 7 days after rash onset,61 viremia generally clears 2 to 3 days after rash onset in parallel with the appearance of antibody.62 Persons with measles are generally considered to be infectious 4 days before through 4 days after rash onset.
Measles virus infection causes simultaneous activation and suppression of the immune system.63–67 Measurement of cytokines released during measles suggests activation of CD8+ T cells, which are important for viral clearance, and type 2 CD4+ T cells, which provide optimal antibody production. Measles virus infection leads to a decrease of CD4+ lymphocyte counts that begins before the onset of rash and lasts for up to 1 month.68 A study of measles patients in The Gambia found marked suppression of the production of interleukin-12, a known regulator of cellular immunity, and suggests a mechanism for the immune suppression associated with measles infection.67 Recovery from infection is associated with the production of serum and secretory antibodies62,69–77 as well as the establishment of cellular immunity.65,66,78–84 Although subclinical infection with boosting of antibody may occur with subsequent exposure,70,72 immunity after natural infection is believed to be lifelong.6,85
Complications
The complications associated with measles infection have been the subject of much description and review.50,52,86–111 Measles illness severity, complication rates, and clinical outcomes vary by the person’s age, preexisting health and nutritional status, comorbidities, access to treatment, and quality of care. In industrialized countries and settings with access to optimal care, the most commonly cited complications associated with measles infection are otitis media (7–9%), pneumonia (1–6%), diarrhea (8%), postinfectious encephalitis (1–4 per 1000–2000 cases of measles), subacute sclerosing panencephalitis (SSPE) (1 per 2500–10,000 measles cases),112 and death (1.0–3.0 per 1000 cases).113 Complications are likely to be present if the fever has not lysed within 1 to 2 days of rash onset. The risk of serious complications and death is increased in children younger than 5 years and adults older than 20 years.2,97,98,102,105 Pneumonia, which is responsible for approximately 60% of deaths, is more common in young patients, whereas acute encephalitis occurs more frequently in adults.97,105 Pneumonia may occur as a primary viral pneumonia (Hecht pneumonia) or as a bacterial superinfection, most commonly with staphylococcus, pneumococcus, or typeable (encapsulated) Haemophilus influenzae. Other described complications include thrombocytopenia, laryngotracheobronchitis, stomatitis, hepatitis, appendicitis and ileocolitis, pericarditis and myocarditis, glomerulonephritis, hypocalcemia, and Stevens-Johnson syndrome.50,52 Based on clinical observations, it has long been assumed that measles infection exacerbates active tuberculosis and activates latent tuberculosis because of the theoretical risk from the measles virus–induced immunosuppression114; however, results from available published studies attempting to determine the occurrence and frequency of this potential additional measles complication are inconclusive.115–117
Measles infection runs a devastating course in children in developing countries and settings with minimal care, where measles mortality rates can be as high as 2% to 15%.2,50,52,110,118–133 The rash is intense and may be hemorrhagic (black measles), and it resolves after marked desquamation. Pneumonia is the most common severe complication from measles and is associated with the greatest number of measles-associated deaths.134,135 Inflammation of the mucosa leads to stomatitis and diarrhea. Diarrhea is a frequent cause of death because it may persist long after the acute insult and further aggravate a preexisting malnourished state.106,136,137 Mediastinal and subcutaneous emphysema, keratitis, corneal ulceration, and gangrene of the extremities are not uncommon. The combination of vitamin A deficiency and keratitis results in a high incidence of blindness.138–140 Secondary bacterial infections, often with staphylococci, produce pustules, furuncles, pneumonia, osteomyelitis, and other pyogenic complications. Neurological complications, including acute viral or bacterial encephalitis, which often result in permanent brain damage, occur in approximately 1 to 4 per 1000 cases.3,56,113,141
Following acute measles virus infection, a persistent infection in the central nervous system tissue with a defective measles virus may occur causing SSPE, a degenerative central nervous system disease that is characterized by behavioral changes, intellectual deterioration, mental and motor deterioration, seizures, and death.141–145 SSPE signs and symptoms begin an average of 7 years after the primary measles infection. Once symptoms start, the person with SSPE develops progressive personality changes, myoclonic seizures and motor disability, and eventually lapses into a coma and dies.146 Because SSPE symptoms are nonspecific and have very late onset after the initial measles infection,147 SSPE cases largely go undetected, particularly in resource-limited settings. The global burden of SSPE is unknown; and the previous estimate of 1 SSPE case per 100,000 measles cases is now thought to have been an underestimation. During the resurgence of measles in the United States during 1989–91, the occurrence of SSPE was estimated to be 22 per 100,000 reported measles cases.144 In Germany, among reported measles cases during 1994–2001, the risk of developing SSPE for children younger than 5 years of age contracting measles infection was estimated to be 1 : 1700 to 1 : 3300.112,144 SSPE incidence depends on the measles epidemiology of a particular setting, in part because the risk for SSPE is higher when the primary measles infection occurs at a younger age,141,148 and among reported SSPE cases, males outnumbered females 2 : 1 to 4 : 1.4,100,104,149 The pathogenesis of measles in SSPE cases is not fully understood; it is thought that measles virus enters the brain, likely during the acute primary measles infection, then persists in the nervous system. Patients with SSPE have high titers of measles-specific antibodies in their sera and cerebrospinal fluid, and measles virus can be detected in affected brain tissue by electron microscopy and polymerase chain reaction (PCR).150 Measles viruses isolated from affected brain tissue of SSPE cases have been found to have critical mutations in M, H, or F genes that prevent normal virus replication and budding from the host membrane. Measles viruses from SSPE cases do not play a role in virus transmission.142,151
Measles infection during pregnancy is associated with an increased risk of maternal, fetal, and newborn complications including miscarriage, preterm birth, neonatal low birth weight, and maternal death.152–156 Because physiological adaptations in the immune system during pregnancy can increase a woman’s susceptibility, pregnant women infected with measles are more likely to be hospitalized, develop pneumonia, and die than nonpregnant women.25,26 Clinical illness in the newborn after intrauterine exposure follows a shortened incubation period and may vary from mild to severe, and fatal.157,158 There is no convincing evidence that maternal infection with measles is associated with congenital malformations.157,159
Exploratory studies of other potential measles-related complications have been conducted. For example, a team of investigators in the United Kingdom has suggested that infection with measles virus or measles vaccine virus may result in a persistent infection of the gastrointestinal tract, leading to Crohn disease or ulcerative colitis later in life.160–163 They proceeded to study a subset of children with developmental disorders and ileocolonic lymphonodular hyperplasia by using reverse transcriptase–PCR (RT-PCR) techniques, and reported finding evidence of measles virus in biopsies from the terminal ileum of these children.164 The findings of this group of investigators have led to speculation that combined vaccination with measles-mumps-rubella (MMR) vaccine may be responsible not only for some forms of inflammatory bowel disease but also the increasing reported incidence of childhood autism. Other researchers, however, have been unable to replicate earlier laboratory findings by using similar or more sensitive and specific methods.165,166 Several subsequent well-conducted epidemiologic studies have found no association between measles vaccination and development of inflammatory bowel disease167–169 or autism.169–171 In fact, the available laboratory and epidemiologic evidence does not support hypotheses that measles or measles-containing vaccines might contribute to or induce inflammatory bowel disease or autism172 (see “Vaccine Safety”). Furthermore, in 2010, the editors of The Lancet retracted the original article that led to the controversy,173 and in January 2011, the British Medical Journal published an investigative review that found the medical records had been incorrectly abstracted to falsely implicate the vaccine.174
Measles has been proposed to be a causal factor for several other diseases. Studies from different laboratories have revealed conflicting evidence for persistence of measles virus in affected tissues from patients with otosclerosis175 and Paget disease.176 Also, current evidence is inconclusive regarding a possible role of measles infections in the pathogenesis of multiple sclerosis.177
Ebolavirus Vaccine History
by Ron Smith, MD | April 9, 2021 | ebolavaccinehistory | 0 Comments
Prior to the 2014 outbreaks of Ebola in west Africa, Ebolavirus disease (EVD or EHF) outbreaks were rural, and through aggressive contact tracing were effectively contained and ended. The source host for this filovirus was then and continues to be unknown.
Then in 2014, the virus got into the urban areas of several west African countries. The epidemic was traced back to an eighteen-month-old boy in Guniea. At that time, Médecins Sans Frontières and Samaritan’s Purse were the only two organizations responding clinically on the ground in the Liberia, Guinea, and Sierra Leone areas. The outbreak was much larger than any before since previous occurrences were rural and not urban. Médecins Sans Frontières and Samaritan’s Purse were taxed to the breaking point and the outbreak in Guinea, Sierra Leone, and Liberia became the largest in history dwarfing all previous ones.
The development of the Ebola vaccine is far from recent and really began in the mid 1990s. Prior that time, vaccines were based on sub-units of the infectious agent. That is, virus or bacteria had to be grown, harvested and only the “active” proteins retrieved which were included in the vaccine. The earlier lessons of the Cutter Incident with the polio vaccine and the high frequency of side effects with the whole cell pertussis vaccine demonstrated the need for a more targeted approach.
In the mid-1990s, Katalin Karikó, a Hungarian-born scientist, pioneered a new approach using mRNA instead of sub-units. Rather than collecting proteins from viruses and bacteria grown in living tissues, her idea was to develop a vaccine using the specific mRNA which coded very specifically for things like the spikes on viruses which attached them to the host cell membrane which then engulfed and “swallowed” the virion. If antibodies to these spike proteins were present already, then virus particles could never attach and never be engulfed by the host cell.
Karikó’s task was to somehow get the mRNA that coded for these spikes into the host cell whereby only the spikes would be produced. Once presented to the immune system, the proper antibody would be made and memorized. Future challenges by the actual virus then would be met swiftly with antibodies attacking the spike proteins preventing infection of the host cell. The University of Wisconsin had already proved this technique worked in mice in 1990.
The problem of creating mRNA was the main issue. Genomic sequencing became partner to all this as sequencing wholesale DNA and RNA strands became possible and very quick. It took only about 48 hours to sequence the mRNA for SARS-CoV-2 in 2019. A second problem with mRNA is how quickly it is degraded and disposed of outside the host cell. A virion with the spike proteins easily carried that mRNA code into the host cell, but simply injecting mRNA into a person won’t do the same.
With the 2014 Ebola outbreak, vaccine development first started using a viral vector to get the mRNA into the host. The idea is to attach the target mRNA to that of another virus which is not lethal and mostly innocuous. That virus would then be the vector by which the host cell would then produce the target spike protein that would in turn lead to antibody production against Ebolavirus.
In the 1990s, John “jack” Rose at Yale used a livestock virus called VSV (vesicular stomatitis virus) as this viral vector. Heinz Feldmann was instrumental in fusing the key Ebola mRNA onto VSV at the Philipps-Universität Marburg in Germany. This vaccine was called rVSV-ZEBOV. He tested it in animals before leaving the Canada Nation Microbiology Laboratory in Winnipeg in 2008. Steven Jones, an associate of his whose name is on the Ebola vaccine patent, began development of human-grade vaccine for the initial testing trials.
As the 2014 Ebola epidemic unfolded, Gary Kobinger, the head of special pathogesn at the National Laboratory in Winnipeg, was watching. His team had been working on the Ebola vaccine for years which showed great promise in animal testing. Vaccine development had gone so far as to produce one ready for human trials. The WHO declined his offer of the vaccine, however, and probably out of Africa’s history of unethical vaccine development by western entities years earlier with polio.
Essentially as a result of the 2014 Ebola outbreak, Merck finally picked up rVSV-ZEBOV, and the first viral vector vaccine for Ebola was branded as Ervebo. The VSV virus vector would go on into research for flu, mealses, SARS, and ZIKA vaccines. The vaccine was initially approved for compassionate use in 2018. It proved highly effective, and was FDA approved in 2019.
In the 2014 epidemic, both Dr. Kent Brantly and Nancy Writebol working for Samaritan’s Purse in Liberia contracted Ebola Zaire (Facing Darkness). This was, of course, before the Ervebo vaccine was available. However, another technology called ZMapp was already in testing and was available for experimental use.
ZMapp developed by Mapp Biopharmaceutical is not a vaccine, but rather three plant-made monoclonal antibodies which attack the Ebola virus. The tobacco plant Nicotiana benthamiana is infected with a viral vector. There are a number of plant viruses that are effective vectors such as tobamoviruses, Potexviruses (e.g. Potato Virus X) , Tobraviruses (e.g., tobacco rattle virus), Geminiviruses (e.g., bean yellow dwarf virus), and Comoviruses (e.g. cowpea mosaic virus).
ZMapp was effective in saving 100% of rhesus macaques infected with Ebola even up to 5 days before administration. Many animals already showed symptoms and had Ebola-driven laboratory aberrations before the drug. The drug was given to both Dr. Brantly and Writebol. They both survived Ebola Zaire. Miguel Pajares, a Spanish priest, received the third course of ZMapp but subsequently died from Ebola.
Viral vector vaccines led to the development of newer mRNA vaccines which have a lipid coating. This coating similarly acts like a virion so that the virus spike protein is manufactured from the mRNA and then released without harming the host cell. The immune cells then process the spike protein and create antibodies to it. Free of the overwhelming assault of viruses like Ebola and SARS-CoV-2, the immune system is equipped to fight the infection without ever having been infected with the pathogen. mRNA vaccines may well signal newer, safer, and more effective measures than many current vaccines.1
Copyright secured by Digiprove © 2021 Ronnie Smith
ENDNOTES
- Historical information sources: ‘Against all odds’: The inside story of how scientists across three continents produced an Ebola vaccine, ZMapp, ↩